Cavitation Studies in Soft Tissue Could Lead to Improved Treatments for Concussion and Other Health Conditions
Cavitation Studies in Soft Tissue Could Lead to Improved Treatments for Concussion and Other Health Conditions
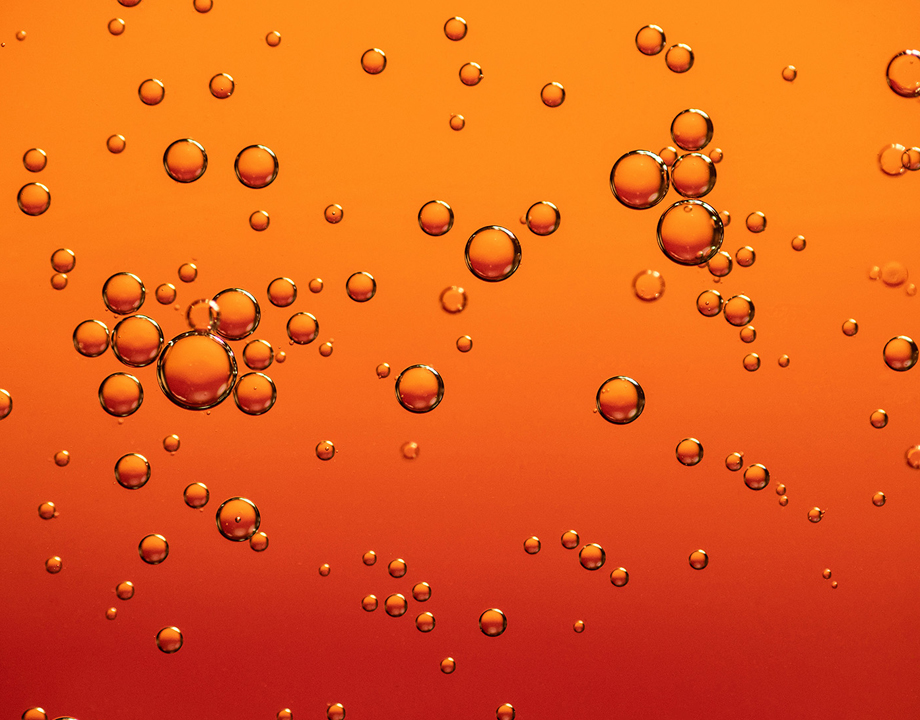
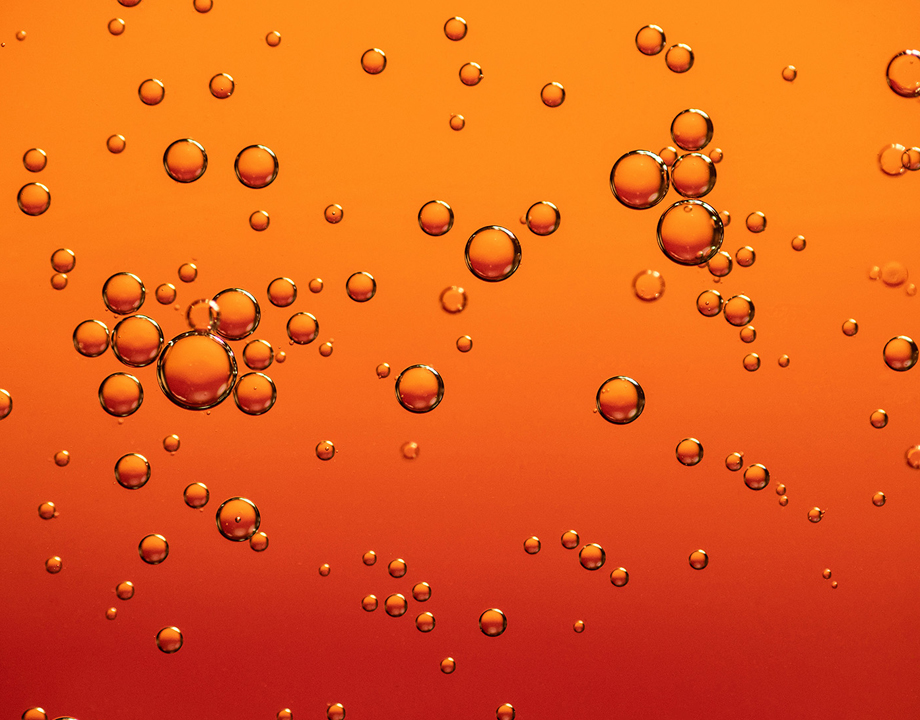
Researchers at Purdue University have developed a theoretical and computational model that describes the dynamics of cavitation bubbles in soft porous materials, such as human tissue.
When a bubble forms in a liquid and then collapses, it generates pressure shock waves that move outward, a process called cavitation.
Cavitation is a common occurrence in the human body. For example, cracking your knuckles is the sound of bubbles popping in the fluid in those joints. A few released and collapsed bubbles are not a big deal, but millions of popping vapor bubbles can cause significant damage to solid rigid structures such marine propellers.
Inside the body, popping vapor bubbles can also damage soft tissues, like with head impacts and concussions. Vapor bubbles form and violently collapse, contributing to brain trauma.
The growth and collapse of cavitation bubbles is a complex process that has been studied for centuries. Past research has focused on the behavior of cavitation bubbles in pure liquids. However, during many daily processes, “cavitation impacts soft porous materials, such as human tissue, and very little is known about these dynamics,” said Hector Gomez, professor of mechanical engineering at Purdue University.
More Like This: Engineers Know How to Have Fun
Cavitation, even in a pure liquid, is a very complex phenomenon that involves non-equilibrium thermodynamics, strong shock waves, fluid dynamics, and many other factors on a scale of micrometers and microseconds.
To learn more about how cavitation behaves in soft porous materials, Gomez and his research team, in collaboration with healthcare engineering professor Pavlos Vlachos at Purdue, developed a computational and mathematical model to describe the dynamics of these cavitation bubbles in a deformable porous medium.
This could have big impacts for the treatment of cavitation-related health conditions such as concussion and drug delivery to tumors.
A hundred years ago, Lord Rayleigh (a British physicist who won the Nobel Prize in 1904) developed the equation that describes the dynamics of a bubble in a liquid. Taking that as his starting point, Gomez eventually modified that equation to describe the bubble dynamics in a soft porous material. “It was surprising that these complex physics still lead to a simple and elegant equation,” said Gomez.
The new model shows that the deformability of a porous material slows the collapse and expansion of cavitation bubbles. The model embedded the bubbles into deformable porous materials, such as the human brain.
Related Content: Microhammer Aids Understanding of Brain Trauma
“The human brain is like a water-filled squishy sponge,” said Vlachos. “It has the consistency of gelatin. The brain material is porous, heterogeneous, and anisotropic, creating a much more complex scenario for experimentation.”
The fact that porous material slows the collapse and expansion of cavitation bubbles, and breaks down the classic scaling relation between bubble size and time, “may have profound consequences for diverse phenomena that involve cavitation in soft porous materials, from traumatic brain injury and drug delivery to spore dispersion,” he added.
Gomez and his collaborators are already planning experiments to physically validate their theoretical results. They are especially interested in the possible applications that could result from this new research.
One potential application is targeted drug delivery in the tumor microenvironment. Killing cancer cells requires high drug doses that can be toxic to other cells in the body. Many drug-delivery systems use encapsulations of the drug to ensure they are released only at the tumor.
“The difficulty is to break the encapsulation at the right location,” said Gomez. “Such encapsulation can be broken by using bubbles. Our research provides a better understanding of how these bubbles collapse in the body and can lead to more effective drug delivery.”
Sign up: ASME Membership Benefits
The research can also be extended to study the impact of uncontrolled cavitation collapse on brain tissue—when military personnel and civilians are exposed to blast shock waves, for example.
Mechanical engineers can also apply the results of this study of cavitation bubbles using engineering principles to their own projects, especially for medical and biological applications.
These findings open new opportunities to understand the complex dynamics of cavitation in soft porous materials, “which may lead to technological advances in medical imaging and drug delivery processes that rely on the violent collapse of cavitation bubbles,” said Gomez. “We look forward to seeing how our team and others researchers can use this knowledge in future research.”
Mark Crawford is technology writer based in Corrales, N.M.
Cavitation is a common occurrence in the human body. For example, cracking your knuckles is the sound of bubbles popping in the fluid in those joints. A few released and collapsed bubbles are not a big deal, but millions of popping vapor bubbles can cause significant damage to solid rigid structures such marine propellers.
Inside the body, popping vapor bubbles can also damage soft tissues, like with head impacts and concussions. Vapor bubbles form and violently collapse, contributing to brain trauma.
The growth and collapse of cavitation bubbles is a complex process that has been studied for centuries. Past research has focused on the behavior of cavitation bubbles in pure liquids. However, during many daily processes, “cavitation impacts soft porous materials, such as human tissue, and very little is known about these dynamics,” said Hector Gomez, professor of mechanical engineering at Purdue University.
More Like This: Engineers Know How to Have Fun
Cavitation, even in a pure liquid, is a very complex phenomenon that involves non-equilibrium thermodynamics, strong shock waves, fluid dynamics, and many other factors on a scale of micrometers and microseconds.
To learn more about how cavitation behaves in soft porous materials, Gomez and his research team, in collaboration with healthcare engineering professor Pavlos Vlachos at Purdue, developed a computational and mathematical model to describe the dynamics of these cavitation bubbles in a deformable porous medium.
This could have big impacts for the treatment of cavitation-related health conditions such as concussion and drug delivery to tumors.
A hundred years ago, Lord Rayleigh (a British physicist who won the Nobel Prize in 1904) developed the equation that describes the dynamics of a bubble in a liquid. Taking that as his starting point, Gomez eventually modified that equation to describe the bubble dynamics in a soft porous material. “It was surprising that these complex physics still lead to a simple and elegant equation,” said Gomez.
The new model shows that the deformability of a porous material slows the collapse and expansion of cavitation bubbles. The model embedded the bubbles into deformable porous materials, such as the human brain.
Related Content: Microhammer Aids Understanding of Brain Trauma
“The human brain is like a water-filled squishy sponge,” said Vlachos. “It has the consistency of gelatin. The brain material is porous, heterogeneous, and anisotropic, creating a much more complex scenario for experimentation.”
The fact that porous material slows the collapse and expansion of cavitation bubbles, and breaks down the classic scaling relation between bubble size and time, “may have profound consequences for diverse phenomena that involve cavitation in soft porous materials, from traumatic brain injury and drug delivery to spore dispersion,” he added.
Gomez and his collaborators are already planning experiments to physically validate their theoretical results. They are especially interested in the possible applications that could result from this new research.
One potential application is targeted drug delivery in the tumor microenvironment. Killing cancer cells requires high drug doses that can be toxic to other cells in the body. Many drug-delivery systems use encapsulations of the drug to ensure they are released only at the tumor.
“The difficulty is to break the encapsulation at the right location,” said Gomez. “Such encapsulation can be broken by using bubbles. Our research provides a better understanding of how these bubbles collapse in the body and can lead to more effective drug delivery.”
Sign up: ASME Membership Benefits
The research can also be extended to study the impact of uncontrolled cavitation collapse on brain tissue—when military personnel and civilians are exposed to blast shock waves, for example.
Mechanical engineers can also apply the results of this study of cavitation bubbles using engineering principles to their own projects, especially for medical and biological applications.
These findings open new opportunities to understand the complex dynamics of cavitation in soft porous materials, “which may lead to technological advances in medical imaging and drug delivery processes that rely on the violent collapse of cavitation bubbles,” said Gomez. “We look forward to seeing how our team and others researchers can use this knowledge in future research.”
Mark Crawford is technology writer based in Corrales, N.M.