Turning Bubbles into Diagnostic Powerhouses
Turning Bubbles into Diagnostic Powerhouses
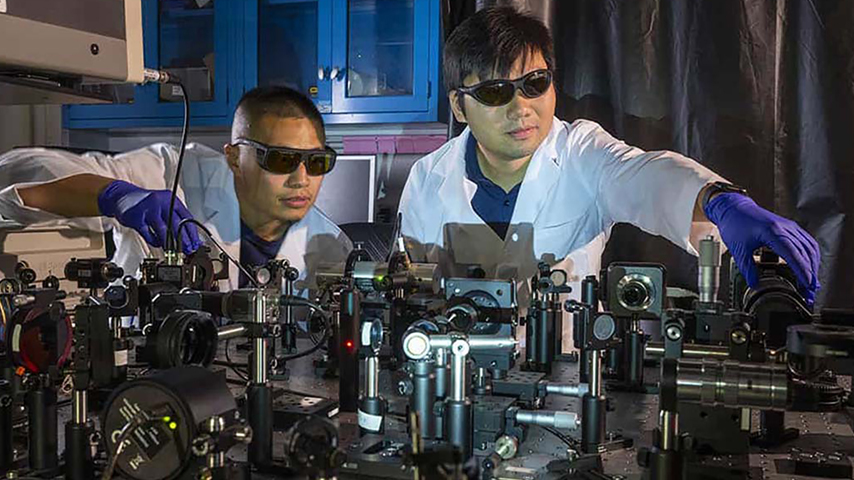
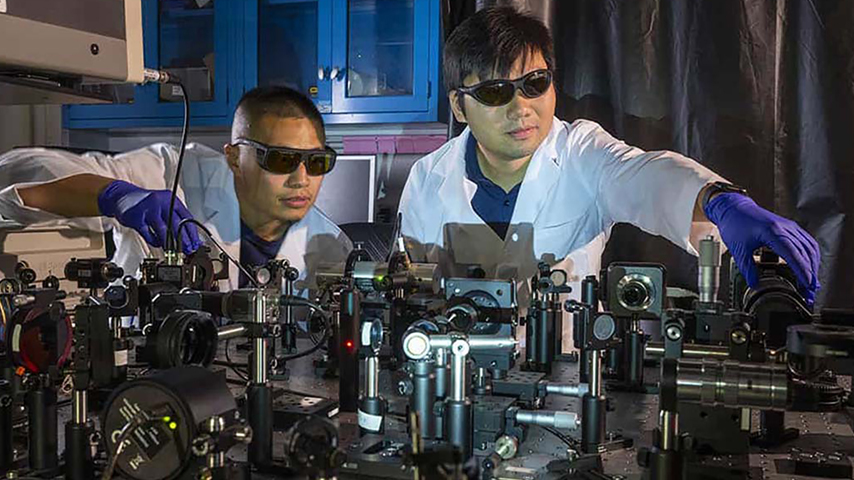
With the help of microgravity, researchers at the University of Notre Dame, are developing an extremely sensitive biosensor.
Is the future of healthcare in space? The 1997 film, Contact, featured a character who moved to a space station as a means to minimize the spread of his cancer. The movie was fiction, but now researchers believe the microgravity environment of orbit may provide a new and powerful venue for detecting cancer and other diseases at a very early stage.
These researchers, led by Tengfei Luo, associate chair of mechanical and aerospace engineering at the University of Notre Dame in Indiana, believe the weightlessness provided by an orbiting spacecraft can facilitate a powerful new form of biosensing.
After studying heat transfer fluid multi-phase problems over the years, Luo discovered that liquid samples, after being heated by a laser, form bubbles—and those bubbles can concentrate different substrates within those liquids.
“These bubbles we can generate collect and concentrate substances that are suspended in the solution,” he explained. “The bubble attracts those substances through the so-called Marangoni flow, which then brings them to the surface of the bubbles.”
The Marangoni effect, a well-known mass transfer phenomenon, is the congregation of molecules that occurs between two phases thanks to a gradient in the surface tension. Luo’s work suggests that the substances collected by the bubbles, which could be cancer-related proteins or trace amounts of nanoplastics pollution, can help congregate markers for easier detection. But optimal collection requires a microgravity environment, where the bubble grow factors bigger than they do on Earth, as well as helps to keep the bubbles tethered to the solution.
“That allows us to collect these markers on the bubble and, once the bubble shrinks and disappears, we have a deposit of the substance in one place,” Luo explained. “Instead of being suspended sparsely in the solution, you can have a high concentration of what you are looking for on a very small spot on the surface.”
But they key is microgravity. Luo said to imagine heating water in a pan or kettle on any home stove top. As the water starts to boil, bubble form, but they “naturally detach” and burst. As a result, any markers that may have been collected on that bubble will disperse. So, in order to support the kind of powerful biosensing application that Luo envisions, you also need the bubbles to stay put.
“We don’t want the bubbles to detach from the surface,” he said. “But that requires microgravity. Because it is the gravity that gives bubbles buoyance and allows the bubbles to escape.”
This kind of biosensor, he said, provides scientists the opportunity for a variety of applications. As noted earlier, clinicians could potentially detect a tumor from a small blood sample – or perhaps even identify proteins to suggest a tumor is developing so that doctors can intervene early. Environmental scientists could better understand the impact of microplastic pollution in large bodies of water with only a few drops of water from the source and, again, act before the contamination becomes harder to manage.
To test the feasibility of such applications, however, Luo and colleagues needed to find ways to send samples to space. They conducted a series of experiments aboard the International Space Station (ISS) to do just that. In the first series of experiments, they had astronaut scientists on board create the bubbles in the liquid solution using a laser. They found that bubbles can grow 10 times larger than what can be grown on Earth, making them ideal for marker collection.
“We believe [microgravity] can be a perfect environment for more sensitive biosensing in the future,” said Luo. “And the more sensitive you can make the sensor, the earlier you can detect diseases or pollution.”
Microgravity-mimicking environments on Earth, unfortunately, are not up to the task of these kind of thermal fluid problems. The key to supporting these kind of applications in the future, Luo said, is to find ways to democratize space travel. He believes the “future prosperity” of the private space industry will make it possible to do so.
“We believe it may be sustainable to send blood samples or other fluid samples to the space station as part of a patient’s annual physical exam,” he said. “Because of the bubbles’ ability to concentrate markers, you only need a very small amount of biofluid for this kind of detection.”
Until that day, Luo and colleagues are working to optimize their technique. While they currently use lasers to create their space bubbles in a single sample, they are looking for ways to generate bubbles in multiple liquid samples in an array.
“We have to make sure that the Marangoni flow is flowing in the right direction so you can still collect the substances on the bubbles,” he said. “That’s what we are pursuing now. The thermofluoid mechanism of these bubbles is very different in the microgravity environment. And if we can keep moving forward, it can potentially lead to step changes in terms of this kind of biosensing technology.”
Kayt Sukel is a technology and business writer in Houston.
These researchers, led by Tengfei Luo, associate chair of mechanical and aerospace engineering at the University of Notre Dame in Indiana, believe the weightlessness provided by an orbiting spacecraft can facilitate a powerful new form of biosensing.
After studying heat transfer fluid multi-phase problems over the years, Luo discovered that liquid samples, after being heated by a laser, form bubbles—and those bubbles can concentrate different substrates within those liquids.
“These bubbles we can generate collect and concentrate substances that are suspended in the solution,” he explained. “The bubble attracts those substances through the so-called Marangoni flow, which then brings them to the surface of the bubbles.”
The Marangoni effect, a well-known mass transfer phenomenon, is the congregation of molecules that occurs between two phases thanks to a gradient in the surface tension. Luo’s work suggests that the substances collected by the bubbles, which could be cancer-related proteins or trace amounts of nanoplastics pollution, can help congregate markers for easier detection. But optimal collection requires a microgravity environment, where the bubble grow factors bigger than they do on Earth, as well as helps to keep the bubbles tethered to the solution.
“That allows us to collect these markers on the bubble and, once the bubble shrinks and disappears, we have a deposit of the substance in one place,” Luo explained. “Instead of being suspended sparsely in the solution, you can have a high concentration of what you are looking for on a very small spot on the surface.”
But they key is microgravity. Luo said to imagine heating water in a pan or kettle on any home stove top. As the water starts to boil, bubble form, but they “naturally detach” and burst. As a result, any markers that may have been collected on that bubble will disperse. So, in order to support the kind of powerful biosensing application that Luo envisions, you also need the bubbles to stay put.
“We don’t want the bubbles to detach from the surface,” he said. “But that requires microgravity. Because it is the gravity that gives bubbles buoyance and allows the bubbles to escape.”
This kind of biosensor, he said, provides scientists the opportunity for a variety of applications. As noted earlier, clinicians could potentially detect a tumor from a small blood sample – or perhaps even identify proteins to suggest a tumor is developing so that doctors can intervene early. Environmental scientists could better understand the impact of microplastic pollution in large bodies of water with only a few drops of water from the source and, again, act before the contamination becomes harder to manage.
To test the feasibility of such applications, however, Luo and colleagues needed to find ways to send samples to space. They conducted a series of experiments aboard the International Space Station (ISS) to do just that. In the first series of experiments, they had astronaut scientists on board create the bubbles in the liquid solution using a laser. They found that bubbles can grow 10 times larger than what can be grown on Earth, making them ideal for marker collection.
“We believe [microgravity] can be a perfect environment for more sensitive biosensing in the future,” said Luo. “And the more sensitive you can make the sensor, the earlier you can detect diseases or pollution.”
Microgravity-mimicking environments on Earth, unfortunately, are not up to the task of these kind of thermal fluid problems. The key to supporting these kind of applications in the future, Luo said, is to find ways to democratize space travel. He believes the “future prosperity” of the private space industry will make it possible to do so.
“We believe it may be sustainable to send blood samples or other fluid samples to the space station as part of a patient’s annual physical exam,” he said. “Because of the bubbles’ ability to concentrate markers, you only need a very small amount of biofluid for this kind of detection.”
Until that day, Luo and colleagues are working to optimize their technique. While they currently use lasers to create their space bubbles in a single sample, they are looking for ways to generate bubbles in multiple liquid samples in an array.
“We have to make sure that the Marangoni flow is flowing in the right direction so you can still collect the substances on the bubbles,” he said. “That’s what we are pursuing now. The thermofluoid mechanism of these bubbles is very different in the microgravity environment. And if we can keep moving forward, it can potentially lead to step changes in terms of this kind of biosensing technology.”
Kayt Sukel is a technology and business writer in Houston.
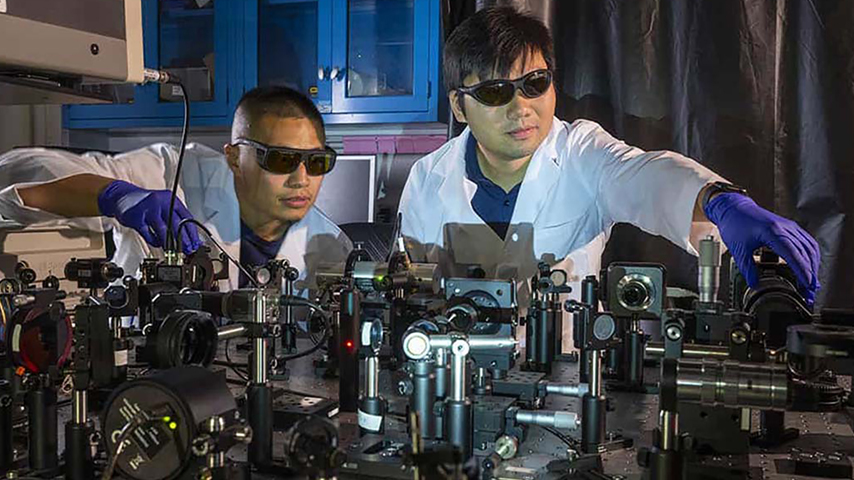
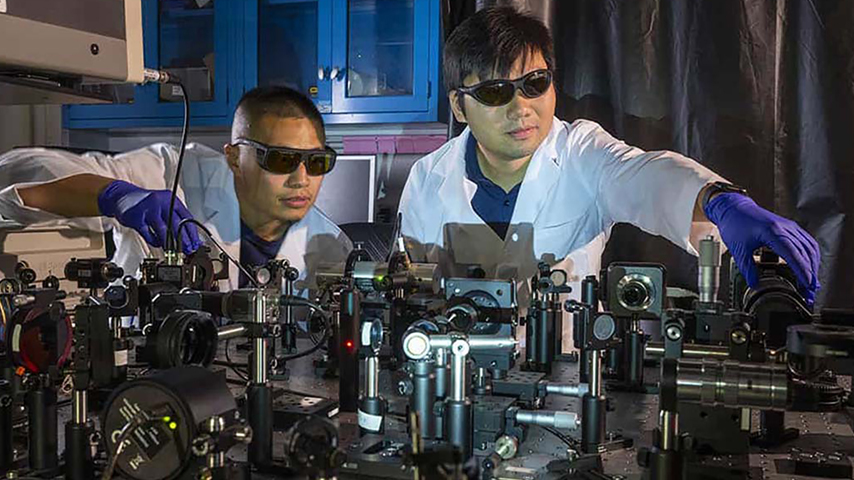