Increasing Sensor Precision with the Seebeck Effect
Increasing Sensor Precision with the Seebeck Effect
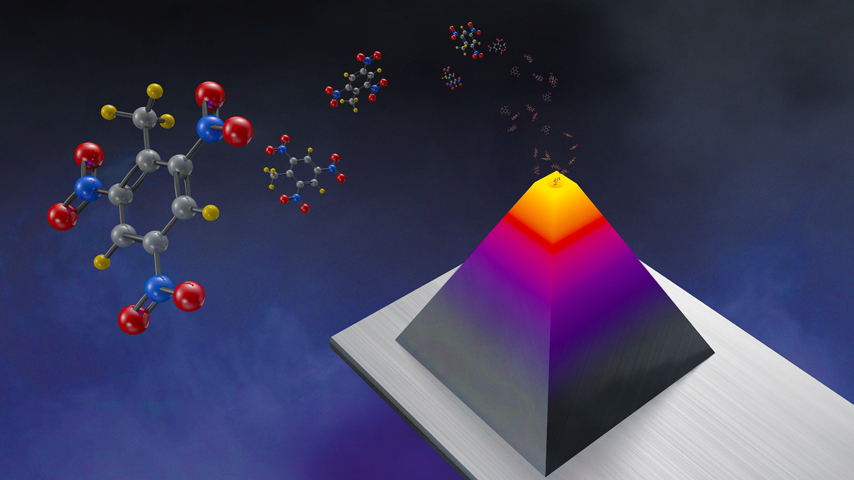
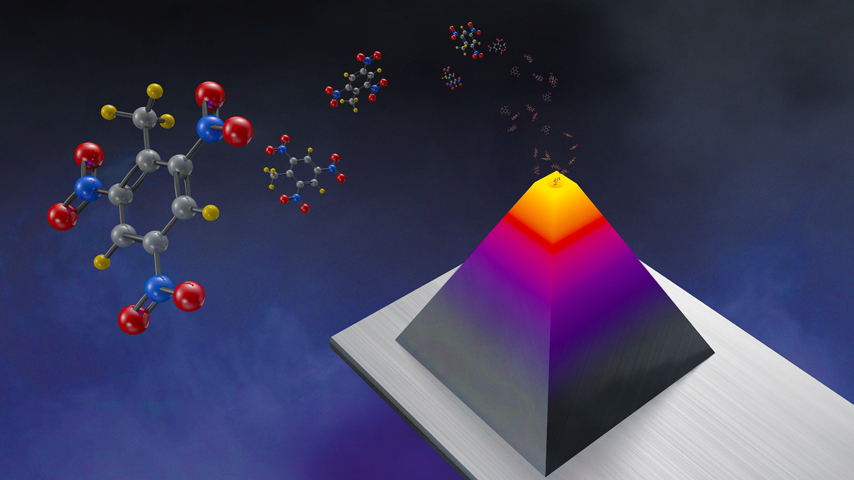
With the help of a thermoelectric phenomenon, researchers can identify molecules down to the attogram, or a quintillionth of a gram, presenting new possibilities for quantum sensor development.
A team with researchers from the U.S. Department of Energy’s Oak Ridge National Laboratory (ORNL) outside of Knoxville, Tenn., and State University of New York (SUNY) at Buffalo have used the more than 200-year-old Seebeck effect to measure otherwise imperceptible molecules.
The Seebeck effect is a thermoelectric phenomenon that describes the voltage resulting from a temperature difference in a circuit made up of two dissimilar electrical conductors when exposed to heat. The team used this technique to identify the heat and light signatures of molecules measured by the attogram—just a quintillionth of a gram. In 1821, German physicist Thomas Johann Seebeck discovered this phenomenon, which has since found widespread use in designing thermocouples, thermoelectric generators, and power plants aimed at converting waste heat to electric power.
The ORNL and SUNY team’s findings are detailed in, “Ultrasensitive Photothermal Spectroscopy: Harnessing the Seebeck Effect for Attogram-Level Detection,” published in Nano Letters.
Microscopic measures
To uncover this new strategy, researchers directed a laser onto a microscopic silicone-based probe, which was in contact with a nanoscale amount of trinitrotoluene (TNT) molecules. The laser “heated” the TNT molecules, which then heated the probe tip, creating a temperature difference between the tip and the reference part of the sensor. This temperature difference generated an amplifiable and measurable voltage used to calculate and confirm the presence of molecules on the sensor.
A temperature difference across a material, or connected conductors, creates voltage as electrons migrate from the hot side to the cold side. The greater the temperature difference, the higher the voltage produced. Materials display differing rates of heat conduction, with some swiftly dissipating heat due to higher thermal conductivity, while others dissipate heat slowly or very little.
“If you illuminate a bunch of molecules with specific colors of light, they get heated and have to do something with that heat. That is called photothermal spectroscopy or photo absorption spectroscopy,” explained Ali Passian a researcher in the Computational Sciences and Engineering Division at ORNL and co-author on the study. “Each molecule type has its likes and dislikes in terms of what colors of light it wants to absorb.”
Become a Member: How to Join ASME
In calculating the Seebeck effect, researchers could establish the relationship between temperature and voltage differences. The sensor materials and their specific Seebeck “properties,” along with the thermal, electrical, and photonic properties of the materials in the nanoscale region of the tip—with and without molecules—need to be considered collectively to compute the sensor response. By encoding the properties of the molecules as they respond to the laser beam into temperature and voltage differences, researchers confirmed a material’s presence.
In real-world applications, the sensor could be used to measure temperature and voltage differences to determine the Seebeck coefficient. However, in the study, the coefficients of the probe materials were known. This allowed researchers to systematically test the process before experimenting with unknown molecules.
The Seebeck effect also functions in reverse, known as the Peltier effect, where the voltage applied across two conductors induces a temperature difference. Heat naturally flows from the hot side to the cold side, without any moving parts, extracting heat from specific materials. French physicist Jean Charles Athanase Peltier discovered this effect in 1834, and it has since been utilized to create thermoelectric cooling devices for refrigeration and temperature control of scientific instruments. Like the Seebeck coefficient, the Peltier coefficient is dependent on the specific characteristics of each conductive material in relation to each other.
Applications
This research has demonstrated promising applications across various fields where detecting small material quantities is crucial. These applications span security, drug enforcement, and smoke detection. For example, fentanyl, a synthetic opioid 50 to 100 times more potent than morphine, is fatal in very small doses. But many sensors lack the sensitivity to accurately identify fentanyl in the small concentrations typically encountered.
“With fentanyl, since you don't need much of it, many sensors fail because there’s just not enough for them to make a good decision,” Passian explained. The implementation of precise sensing equipment could accurately detect the presence of fentanyl in samples, potentially increasing detection frequency and reducing overdoses.
Editor's choice: Better Flaw Detection During Powder Bed Fusion
While this study concentrated on measuring very small quantities of specific molecules, this technology also proved capable of detecting minute changes in quantity.
“It's not just about detection of very few molecules, but also small changes in how many molecules there are,” Passian said. For instance, the amount of methane in relation to the volume of the atmosphere fluctuates continuously. Due to the limited sensitivity of existing sensors, miniscule changes in methane quantity are undetectable until the amount reaches the sensor’s threshold. By enhancing the sensor’s sensitivity, scientists could detect changes in much smaller increments, thereby obtaining more data to formulate precise predictions and detailed recommendations.
With a combination of existing spectroscopy methods and the Seebeck effect, this breakthrough holds great promise for a wide range of applications in sensing trace amounts of molecules or small changes in quantity.
As research in this field continues to evolve, the integration of the Seebeck effect with photothermal spectroscopy promises to revolutionize molecular sensing capabilities and deepen the understanding of complex systems in various scientific disciplines.
Nicole Imeson is an engineer and writer in Calgary, Alberta.