Mechanical Behavior of Coronaviruses Affects Their Infectivity, Lethality
Mechanical Behavior of Coronaviruses Affects Their Infectivity, Lethality
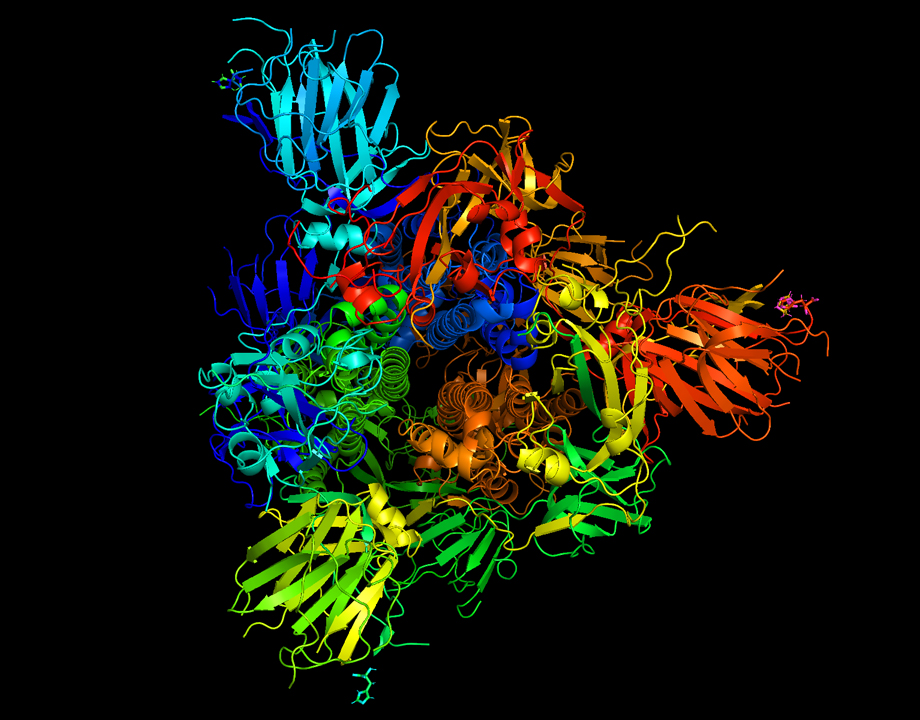
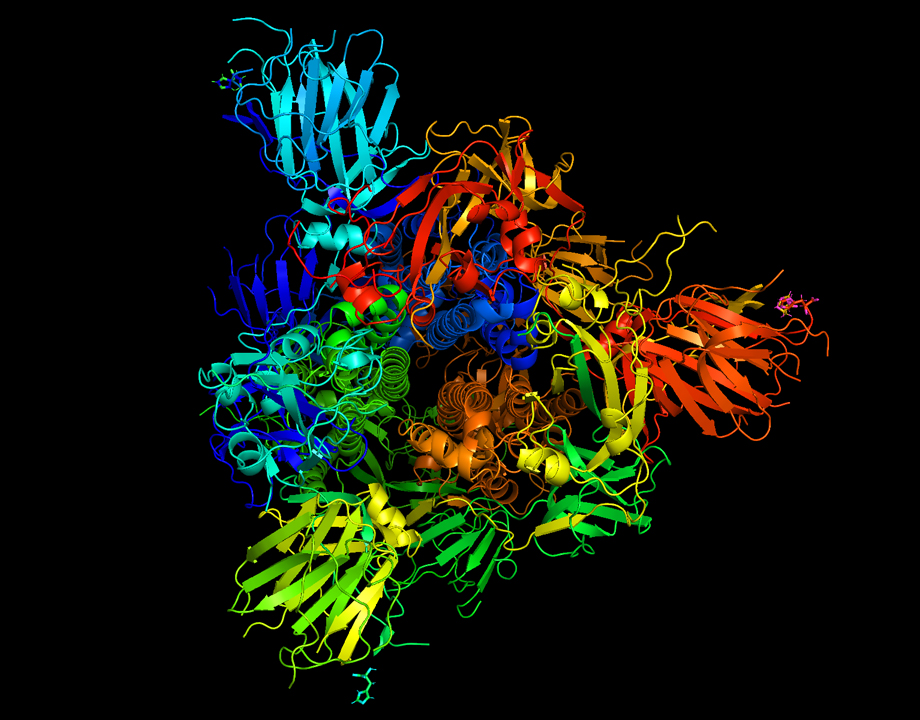
Virus changes shape to enter cells. Image: Markus Buehler and Yiwen Hu, MIT
A study of the mechanical properties of coronaviruses explains how their constant vibrations and shape-changing behavior can successfully infect human cells. Conducted at the Massachusetts Institute of Technology by doctoral student Yiwen Hu and Markus J. Buehler, the McAfee Professor of Engineering, the findings have ramifications in determining the infectiousness and lethality of future coronavirus mutations. In addition, dampening the virus’s vibrations might be one way of denying its access to the human cell, and preventing infections.
The SARS-CoV-2 virus, which causes COVID-19, belongs to a family of coronaviruses, which have spike proteins characterized by the crown-like halo. Spike proteins infect human cells by finding a receptor called ACE2 on which to attach. Much like turning a doorknob to enter a house, once the virus grasps this receptor, it can turn the key and enter the cell, release its genetic material and reprogram the cell to replicate the virus.
The problem: The virus and the receptor don’t fit perfectly every time. But Hu and Buehler found that in cases of imperfect fit, the coronavirus has a way of circumventing the problem: It constantly changes shape slightly so there are greater chances of a fit between virus and receptor, much like jiggling the key in a lock, to find a way in.
Hu said the team has studied the viruses that cause MERS, SARS and COVID-19 in this context and found they all exhibit similar behavior.
Traditional studies of viruses usually focus on their biochemical properties. “We know the virus’s chemical formula, the DNA sequence, but we don’t know much about its mechanical properties,” Buehler said.
“When you think about molecules and chemistry you see these stick figures and think that’s what these objects look like. You imagine them as static structures when they’re actually flexible, you can bend them, they’re mechanical structures,” he said. “There’s a lot that mechanical engineers can offer to [the medical] community.”
Hu and Buehler performed atomistic modeling simulations to focus on the mechanical aspects of the viruses. The small size—SARS-CoV-2 virus is 60-90 nm—makes experimental observations difficult, especially when considering transient, dynamical behavior such as the detailed molecular motions of the molecules that make up the virus.
The study found that the higher the rate and intensity of the coronavirus’s spike’s vibrations, the easier the path to cell entry and the higher the fatality rate. The findings point to the importance of studying the mechanics of viruses for a more complete picture of their behavior.
Two primary mechanical behavior parameters dictate virus infectivity and lethality: variations in the upward swing of one branch of the protein molecule and the ratio between two different conformations of the molecule. The team plotted these two parameters and developed a model by plugging in epidemiological data from coronavirus outbreaks. An increase in these factors dictates the virus’s epidemiological characteristics, making it more lethal and less infectious. MERS is most lethal while the SARS-CoV-2, which causes COVID-19, is extremely infectious and less lethal (see chart).
If the vibrations of the spikes increase the chances of cell infection, then dampening these vibrations or stiffening them in some capacity could form one approach to treating related diseases such as COVID-19. Essentially you want to figure out a way of moving from the top right toward the left of the chart so that the virus becomes less infectious and less lethal.
More on the Fight Against COVID-19: Virus-Vanquishing Surface to Reduce Transmission
Such analysis is significant when studying future mutations of the viruses as simply studying the mechanics might give scientists clues about how fast the virus will spread and how lethal it might be. “The lethality of a virus can be related to the nano-mechanical features. That’s an incredible observation. If there’s an outbreak somewhere, this tool can be used to quickly make an estimate by studying the [mutation] behavior. If it’s found to have greater lethality or greater infectiousness we could take proper public health measures,” Buehler said. “We’re defining our own kind of engineering problem.”
Future work will focus on more mutations, Hu said. “We’re looking at a lot of new mutations to predict how they will behave and also study the effect of temperature,” she noted.
Poornima Apte is an independent writer based in Walpole, Mass.
The SARS-CoV-2 virus, which causes COVID-19, belongs to a family of coronaviruses, which have spike proteins characterized by the crown-like halo. Spike proteins infect human cells by finding a receptor called ACE2 on which to attach. Much like turning a doorknob to enter a house, once the virus grasps this receptor, it can turn the key and enter the cell, release its genetic material and reprogram the cell to replicate the virus.
The problem: The virus and the receptor don’t fit perfectly every time. But Hu and Buehler found that in cases of imperfect fit, the coronavirus has a way of circumventing the problem: It constantly changes shape slightly so there are greater chances of a fit between virus and receptor, much like jiggling the key in a lock, to find a way in.
Hu said the team has studied the viruses that cause MERS, SARS and COVID-19 in this context and found they all exhibit similar behavior.
Traditional studies of viruses usually focus on their biochemical properties. “We know the virus’s chemical formula, the DNA sequence, but we don’t know much about its mechanical properties,” Buehler said.
“When you think about molecules and chemistry you see these stick figures and think that’s what these objects look like. You imagine them as static structures when they’re actually flexible, you can bend them, they’re mechanical structures,” he said. “There’s a lot that mechanical engineers can offer to [the medical] community.”
Hu and Buehler performed atomistic modeling simulations to focus on the mechanical aspects of the viruses. The small size—SARS-CoV-2 virus is 60-90 nm—makes experimental observations difficult, especially when considering transient, dynamical behavior such as the detailed molecular motions of the molecules that make up the virus.
The study found that the higher the rate and intensity of the coronavirus’s spike’s vibrations, the easier the path to cell entry and the higher the fatality rate. The findings point to the importance of studying the mechanics of viruses for a more complete picture of their behavior.
Two primary mechanical behavior parameters dictate virus infectivity and lethality: variations in the upward swing of one branch of the protein molecule and the ratio between two different conformations of the molecule. The team plotted these two parameters and developed a model by plugging in epidemiological data from coronavirus outbreaks. An increase in these factors dictates the virus’s epidemiological characteristics, making it more lethal and less infectious. MERS is most lethal while the SARS-CoV-2, which causes COVID-19, is extremely infectious and less lethal (see chart).
If the vibrations of the spikes increase the chances of cell infection, then dampening these vibrations or stiffening them in some capacity could form one approach to treating related diseases such as COVID-19. Essentially you want to figure out a way of moving from the top right toward the left of the chart so that the virus becomes less infectious and less lethal.
More on the Fight Against COVID-19: Virus-Vanquishing Surface to Reduce Transmission
Such analysis is significant when studying future mutations of the viruses as simply studying the mechanics might give scientists clues about how fast the virus will spread and how lethal it might be. “The lethality of a virus can be related to the nano-mechanical features. That’s an incredible observation. If there’s an outbreak somewhere, this tool can be used to quickly make an estimate by studying the [mutation] behavior. If it’s found to have greater lethality or greater infectiousness we could take proper public health measures,” Buehler said. “We’re defining our own kind of engineering problem.”
Future work will focus on more mutations, Hu said. “We’re looking at a lot of new mutations to predict how they will behave and also study the effect of temperature,” she noted.
Poornima Apte is an independent writer based in Walpole, Mass.
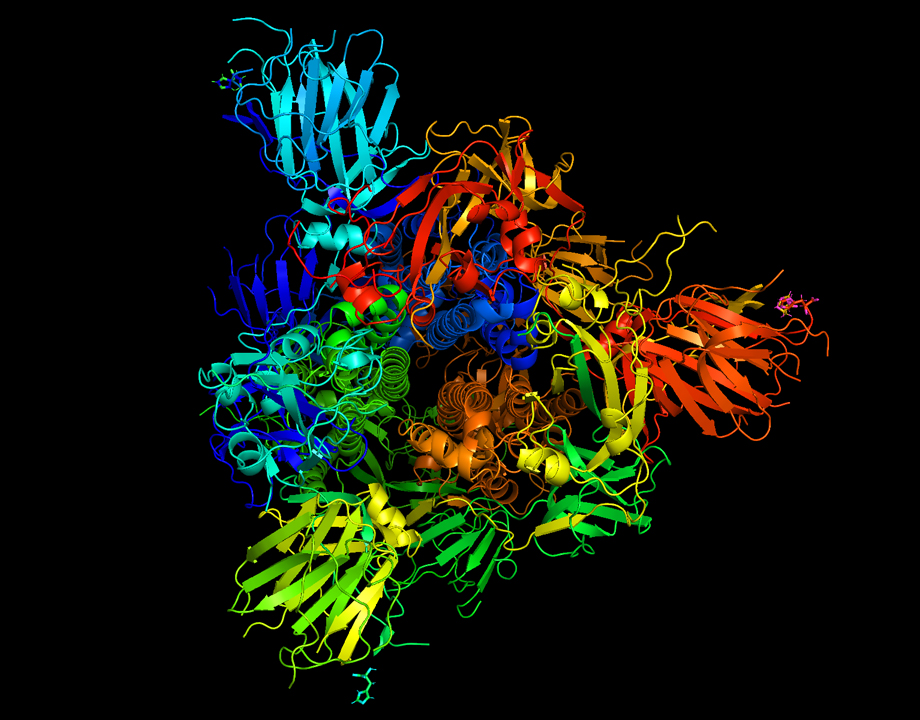
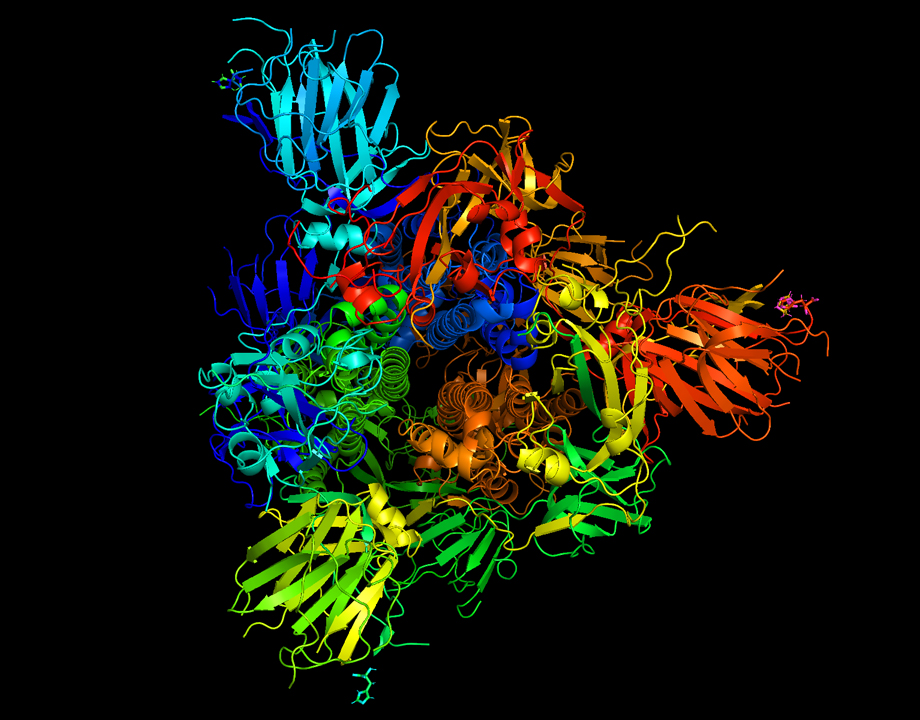