Membrane Could Be Key to Carbon Sequestration
Membrane Could Be Key to Carbon Sequestration
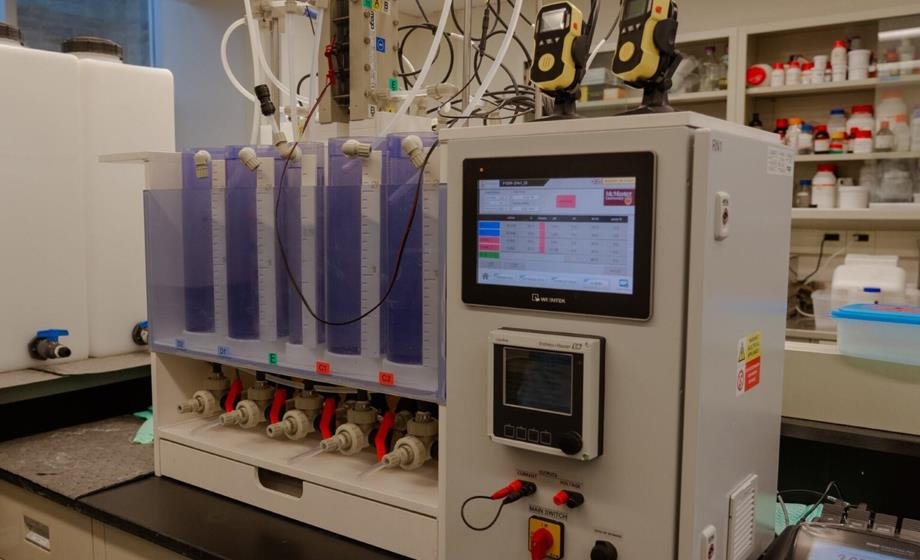
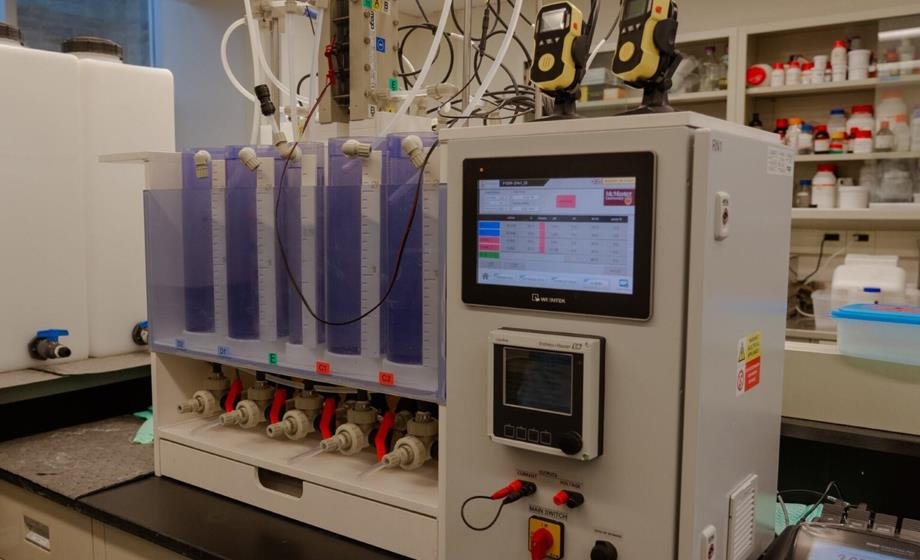
A process that pulls hydrochloric acid from seawater could reduce levels of carbon dioxide in the atmosphere.
The increasing concentration of carbon dioxide (CO2) in the atmosphere is one of the main drivers of climate change, and one that isn’t going away. Even if greenhouse gas emissions ceased today, CO2 levels would remain high and take thousands of years to return to pre-industrial levels. Scientists and industries worked to reduce emissions at the source, but these efforts alone were not enough.
What is needed is a way to pull the excess CO2 from the atmosphere. Researchers at McMaster University in Hamilton, Ont., and the University of Toronto, are looking to take a natural process and accelerate it.
“The oceans are already a massive sink for CO2. They absorb about 30 percent of the excess CO2 in our atmosphere,” said Charles-François de Lannoy, professor of chemical engineering at McMaster.
Seawater is slightly basic, with its average pH measuring 8.1. (7 is completely neutral.) Carbon dioxide can dissolve in seawater to form bicarbonate ions and hydrogen ions, which are acidic. In fact, the average ocean pH has decreased from 8.2 since pre-industrial times, which due to the non-linear nature of pH measurements means seawater is 25 percent more acidic.
Unfortunately, not only is acidic seawater harmful for marine life, but it has a reduced capacity to absorb additional CO2.
When the researchers were looking for an inexpensive and scalable way of offsetting the acidity of seawater, they turned to a market-ready process: bipolar membrane electrodialysis (BMED). An electric field across the membrane enables the separation of positive and negative ions.
“Bipolar membrane electrodialysis is a membrane-based technology that is used to split salts into acids and bases. It's not a new technology, but it hasn't really found too much of a commercial niche,” explained Jay Werber, assistant professor of chemical engineering and applied chemistry at the University of Toronto.
“The bipolar membrane is typically a three-layer structure,” Werber said. “There is a polymer layer with fixed negative charges, a catalyst layer that’s typically spray coated with inorganic nanoparticles, and then another layer with fixed positive charges.”
Energy Blog: Carbon, Carbon Everywhere
The membranes are used for desalination, food processing, and other applications where producing ions in water are important. Bipolar membrane development also has emerged as a significant field among researchers and industry leaders. New applications for bipolar membranes include producing fuel, formic acid, or other chemicals as part of CO2 electrolyzers. In some cases, manufacturers recycled their otherwise wasted byproducts using BMED and reused them for future production.
Even so, industry’s reluctance to adopt bipolar membranes kept prices high and efficiency low. A square meter of bipolar membrane could cost up to $1,000, contrasting sharply with the $20 cost of a square meter of reverse osmosis membrane.
For the work on carbon sequestration, the goal was to decrease the acidity of seawater.
Using BMED, the researchers applied a strong electric current across membrane compartments full of seawater—the saltier the better. This process dissociated H2O into positive hydrogen ions and negative hydroxide ions, which were pulled apart and separated by cation-exchange (negatively charged) and anion-exchange (positively charged) membranes.
The hydrogen ions combined with chloride from dissolved salt to form hydrochloric acid, which was removed, and purified or chemically modified. The chemical has a market value.
Discover the Benefits of ASME Membership
The hydroxide ions combined with sodium ions to form sodium hydroxide, to increase pH and decrease acidity before returning it to the ocean. The full process can enhance the ocean’s ability to absorb more CO2 and increase ocean pH closer to pre-industrial levels.
“The sodium hydroxide added to seawater raises pH and allows CO2 to dissolve into it and turn into carbonates. You're constantly equilibrating with the CO2 in the atmosphere as more CO2 is being pulled out of the atmosphere into the oceans,” de Lannoy said.
“Usually when you scale things up, other challenges arise and we have to figure out what kind of changes we would have to make to maintain the performance,” said Bassel Abdelkader, a postdoctoral researcher at McMaster.
Theoretically, enough CO2 could be removed to offset current carbon emissions and restore pH, but this would require enormous investment and several large-scale installations of the BMED process. For instance, desalination or nuclear power plants applications were explored, where existing infrastructure and increased brine salinity reduced costs and energy for the process. Ships crossing the ocean, already desalinating seawater for onboard consumption, could also use this technology.
More on This Topic: New Hydrogel Removes Carbon Dioxide from Ambient Air
Researchers balanced energy consumption, membrane longevity, and processing time.
“Operating at higher concentrations speeds up the process and captures more carbon dioxide quickly but degrades the membrane faster. We’re optimizing for the best conditions to ensure long-term membrane stability,” Abdelkader said.
The bipolar membranes on the market remained relatively thick, requiring a strong electric field to separate the chemical compounds, making them inefficient. If researchers reduced the membrane thickness, the electric field required would also decrease, lowering the power needed for the process, increasing efficiency, and improving membrane longevity.
Researchers have also aimed to leverage the established production methods of reverse osmosis membranes to drive down the cost of bipolar membranes, which deteriorated faster in the field than their counterparts due to the reactive nature of the electrochemical cells in the electrodialysis processes. Despite membrane costs, “the big driver for using this technology at all is that it's the most energy efficient way you can make a dilute acid and base,” Werber said.
Nicole Imeson is a technology writer in Calgary, Alta.
What is needed is a way to pull the excess CO2 from the atmosphere. Researchers at McMaster University in Hamilton, Ont., and the University of Toronto, are looking to take a natural process and accelerate it.
“The oceans are already a massive sink for CO2. They absorb about 30 percent of the excess CO2 in our atmosphere,” said Charles-François de Lannoy, professor of chemical engineering at McMaster.
Seawater is slightly basic, with its average pH measuring 8.1. (7 is completely neutral.) Carbon dioxide can dissolve in seawater to form bicarbonate ions and hydrogen ions, which are acidic. In fact, the average ocean pH has decreased from 8.2 since pre-industrial times, which due to the non-linear nature of pH measurements means seawater is 25 percent more acidic.
Unfortunately, not only is acidic seawater harmful for marine life, but it has a reduced capacity to absorb additional CO2.
When the researchers were looking for an inexpensive and scalable way of offsetting the acidity of seawater, they turned to a market-ready process: bipolar membrane electrodialysis (BMED). An electric field across the membrane enables the separation of positive and negative ions.
“Bipolar membrane electrodialysis is a membrane-based technology that is used to split salts into acids and bases. It's not a new technology, but it hasn't really found too much of a commercial niche,” explained Jay Werber, assistant professor of chemical engineering and applied chemistry at the University of Toronto.
“The bipolar membrane is typically a three-layer structure,” Werber said. “There is a polymer layer with fixed negative charges, a catalyst layer that’s typically spray coated with inorganic nanoparticles, and then another layer with fixed positive charges.”
Energy Blog: Carbon, Carbon Everywhere
The membranes are used for desalination, food processing, and other applications where producing ions in water are important. Bipolar membrane development also has emerged as a significant field among researchers and industry leaders. New applications for bipolar membranes include producing fuel, formic acid, or other chemicals as part of CO2 electrolyzers. In some cases, manufacturers recycled their otherwise wasted byproducts using BMED and reused them for future production.
Even so, industry’s reluctance to adopt bipolar membranes kept prices high and efficiency low. A square meter of bipolar membrane could cost up to $1,000, contrasting sharply with the $20 cost of a square meter of reverse osmosis membrane.
For the work on carbon sequestration, the goal was to decrease the acidity of seawater.
Using BMED, the researchers applied a strong electric current across membrane compartments full of seawater—the saltier the better. This process dissociated H2O into positive hydrogen ions and negative hydroxide ions, which were pulled apart and separated by cation-exchange (negatively charged) and anion-exchange (positively charged) membranes.
The hydrogen ions combined with chloride from dissolved salt to form hydrochloric acid, which was removed, and purified or chemically modified. The chemical has a market value.
Discover the Benefits of ASME Membership
The hydroxide ions combined with sodium ions to form sodium hydroxide, to increase pH and decrease acidity before returning it to the ocean. The full process can enhance the ocean’s ability to absorb more CO2 and increase ocean pH closer to pre-industrial levels.
“The sodium hydroxide added to seawater raises pH and allows CO2 to dissolve into it and turn into carbonates. You're constantly equilibrating with the CO2 in the atmosphere as more CO2 is being pulled out of the atmosphere into the oceans,” de Lannoy said.
Scaling up
Researchers worked to determine the scalability of their setup, assessing whether the process scales linearly and identifying necessary adjustments for real-world applications.“Usually when you scale things up, other challenges arise and we have to figure out what kind of changes we would have to make to maintain the performance,” said Bassel Abdelkader, a postdoctoral researcher at McMaster.
Theoretically, enough CO2 could be removed to offset current carbon emissions and restore pH, but this would require enormous investment and several large-scale installations of the BMED process. For instance, desalination or nuclear power plants applications were explored, where existing infrastructure and increased brine salinity reduced costs and energy for the process. Ships crossing the ocean, already desalinating seawater for onboard consumption, could also use this technology.
More on This Topic: New Hydrogel Removes Carbon Dioxide from Ambient Air
Researchers balanced energy consumption, membrane longevity, and processing time.
“Operating at higher concentrations speeds up the process and captures more carbon dioxide quickly but degrades the membrane faster. We’re optimizing for the best conditions to ensure long-term membrane stability,” Abdelkader said.
The bipolar membranes on the market remained relatively thick, requiring a strong electric field to separate the chemical compounds, making them inefficient. If researchers reduced the membrane thickness, the electric field required would also decrease, lowering the power needed for the process, increasing efficiency, and improving membrane longevity.
Researchers have also aimed to leverage the established production methods of reverse osmosis membranes to drive down the cost of bipolar membranes, which deteriorated faster in the field than their counterparts due to the reactive nature of the electrochemical cells in the electrodialysis processes. Despite membrane costs, “the big driver for using this technology at all is that it's the most energy efficient way you can make a dilute acid and base,” Werber said.
Nicole Imeson is a technology writer in Calgary, Alta.