Modeling the Miraculous
Modeling the Miraculous
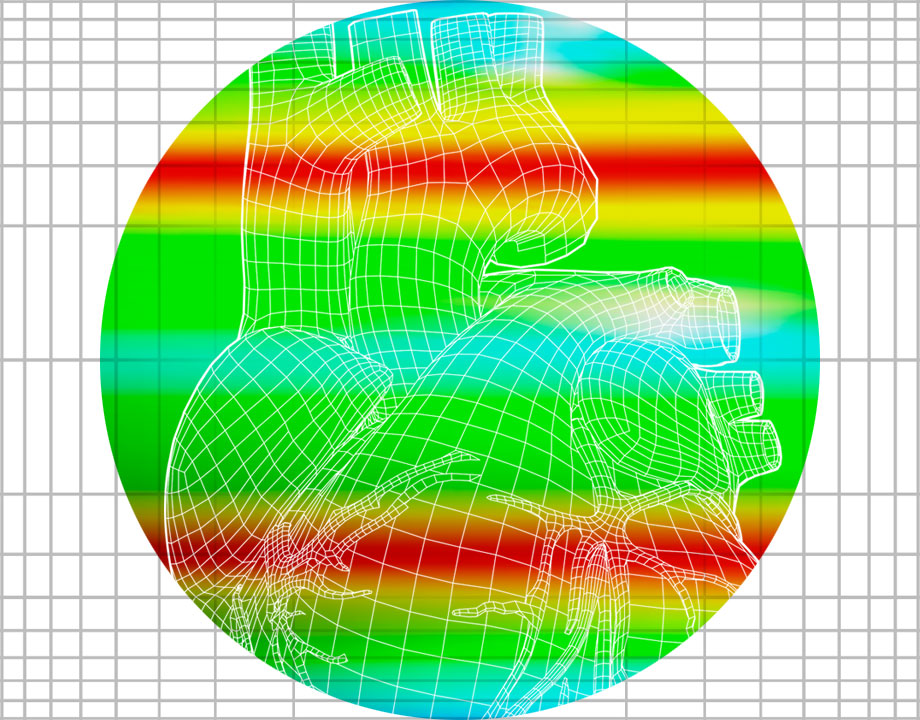
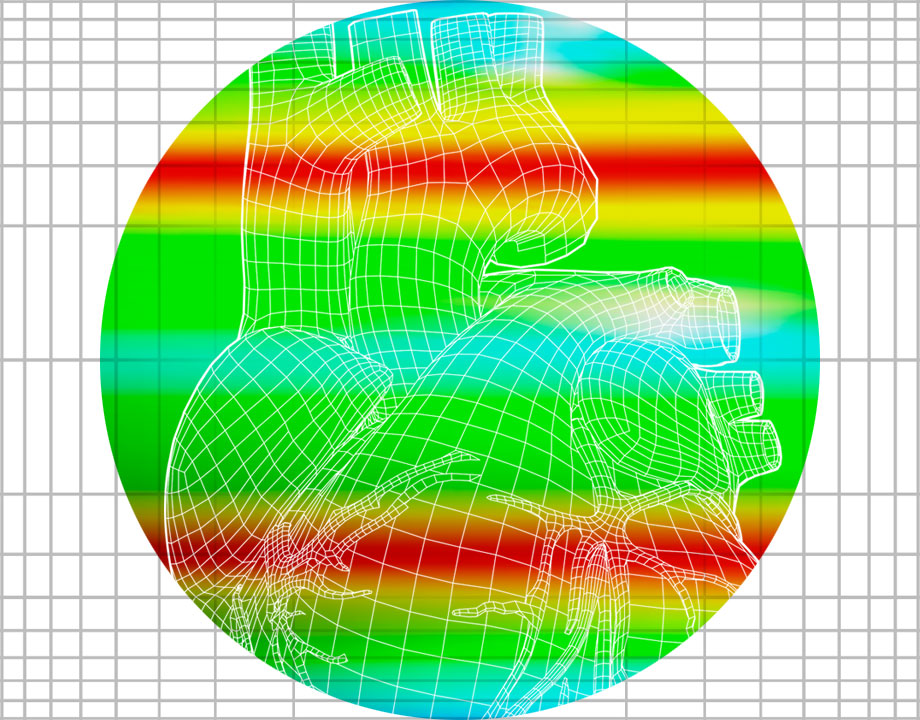
The flow of blood through the human cardiovascular system is complex and not fully understood, some even say miraculous. Buried beneath the skin, feeding our vitals, many properties of our veins and arteries remain hidden from investigating, scientific minds.
But those secrets can be revealed when the tubes of our oxygen dissemination system are well modelled. That’s just what Mohammadali Sharzehee, a doctoral student in the University of Texas at San Antonio’s Department of Mechanical Engineering, has been working at. With his computational models, sufferers of various cardiovascular diseases may soon see their lives improve.
Sharzehee turned his attention from the mechanics of hardware to the mechanics of flesh and blood while working on his bachelor’s thesis at Shiraz University in Iran.
“I found bioengineering to be very well in line with my intellectual curiosity,” he said. “I am passionate about conducting interdisciplinary research and tackling its challenges to make scientific breakthroughs.”
At UTSA, Sharzehee began to look into “vascular tortuosity,” the curves, kinks and loops that our blood vessels can find themselves in, as well as aneurysms. These deformations can restrict blood flow and lead to ischemic attacks—most commonly, a stroke.
Learn more about a Mini Kidney on a 3D-Printed Chip
Arteries that are twisted and have an aneurysm are at a greater risk of rupturing. But the mechanics of exactly why blood vessels become tortuous—or aneurysmal—remained unclear. Sharzehee decided to tackle the problem with computational modeling.
With data gathered from measuring the carotid arteries of pigs, Sharzehee was able to compare simulation results with experimental and theoretical results.
“We found that arteries with aneurysms are more prone to become tortuous, and tortuous shape increases stress in aneurysm,” he said. And his results can be applied beyond the cardiovascular to any tubular organ including the stomach and the esophagus.
In another study—soon to be published—Sharzehee looked at how tortuous vessels form in the first place. The results will help doctors determine how best to treat patients, which may include improved stents and preventative surgery.
“Our model can be used to improve vascular implant design by considering torsional and bending stability,” Sharzehee said. “The location of high mechanical stress can be determined, but the appropriate time for surgical interventions depends on patients.”
Sharzehee is currently studying how myocardial bridge—a condition where a segment of the coronary artery tunnels into cardiac muscle—changes blood flow, as well as its role in the development of atherosclerosis. The project “will examine patients with various level of myocardial bridge to better understand its effects and provide inputs for clinical treatments,” he said.
Editor’s Pick: 6 Ways Engineers Heal a Broken Heart
The work of modeling the cardiovascular system does not always flow uninterrupted. At the start of his research, Sharzehee’s advisor warned him that the complexity and high computational cost of the problem would make the modeling difficult.
“Throughout my computational modeling, I have faced a number of difficulties related to numerical simulations,” Sharzehee said. “For example, I have worked for months without getting any results and felt disappointed with my progress. But, believing in my own capabilities and working with knowledgeable advisors are the two primary factors that made me succeed.”
Michael Abrams is a technology writer based in Westfield, NJ.