Seeing Sponges Through a New Lens
Seeing Sponges Through a New Lens
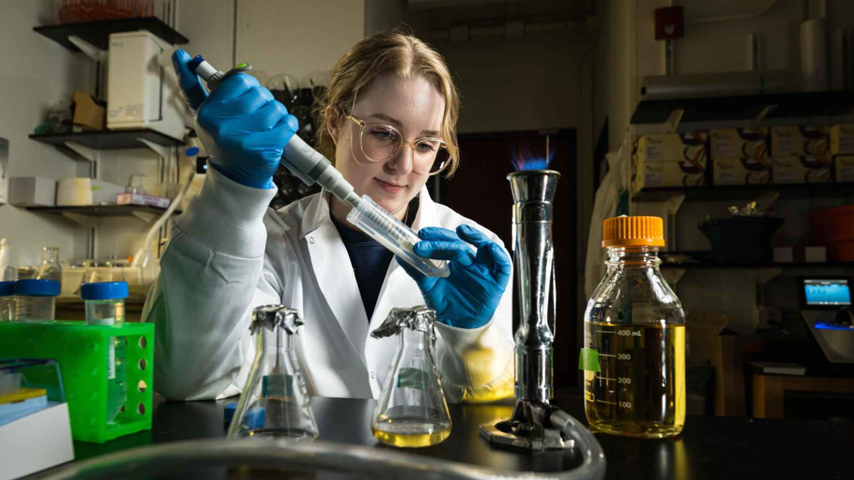
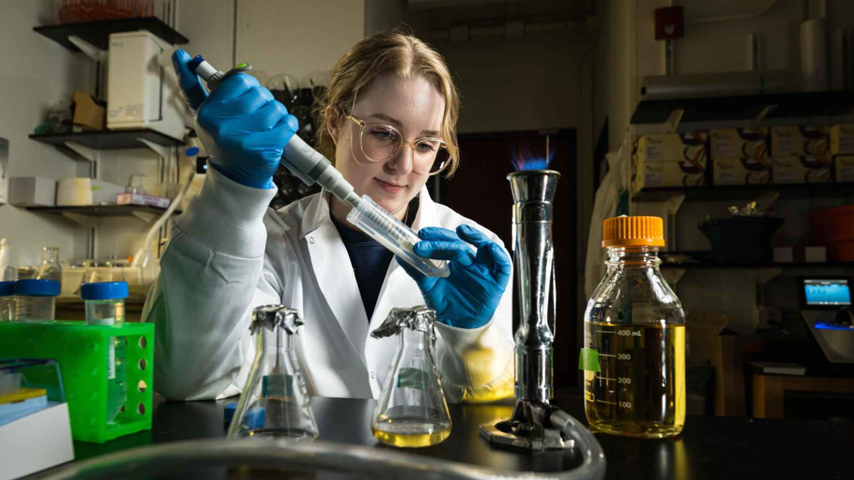
A multi-disciplinary, multi-institutional group is leveraging sea sponge silica to create a new class of bioinspired microlenses.
More than a decade ago, Anne S. Meyer, a biologist at the University of Rochester, met a scientist who was doing captivating work on a type of sea sponge that could mineralize silica, or bioglass, to provide the structure for its underlying skeletal structure.
“I had never heard of [these sponges] before. But the idea that they could make this amazing, mineralized glass material and do it with one single enzyme called silicatein was very inspiring,” she said.
There are many interesting materials that exist in nature that Meyer would love to make using a genetic engineering approach. Yet, most of those materials are quite complex, requiring multiple enzymes and tissues to work together, sometimes in rather mysterious ways, to create the end product. After learning about the sea sponges and the single enzyme required to make silica, she thought it would be an interesting test case for a genetically engineered material—and the intricate glass produced, she hypothesized, could be leveraged to make microlenses, or lenses just a few micrometers in size, with the optical power to capture, focus, and manipulate light.
“It seemed to me that this would make an excellent synthetic biology project,” Meyer said. “And I wondered if it was something it would be possible to engineer bacteria to do.”
Current microlenses are only a few microns in size. They have the power to focus light on a microscopic scale, allowing precise manipulation of beams to support high-resolution imaging for camera sensors, fiber optics, and medical devices.
To see whether they could genetically engineer a biological microlens, Meyer recruited colleagues from the University of Colorado-Boulder, Delft University of Technology, and Leiden University, with collected expertise in optics, physics, and chemistry.
More for You: Big Promise for Largest Metalens
“We used a variety of E. coli that’s not a pathogen and doesn’t cause diseases or have toxic effects,” she said. “And we used it because this is a type of bacteria that is very well studied and characterized. There are a lot of genetic tools already available you can use to express new enzymes and new genetic parts in different parts of the cell.”
After conducting physics modeling in computer simulations, the group determined, in theory, engineering something the size and shape of the bacteria should produce a distinct silica shell with lens-type behaviors. With those tests to guide them, the team was inspired to bring this novel idea into the real world. They grew the engineered cells and checked for the presence of silica on each by analyzing each bacteria’s chemical properties. They then created mathematical models to better understand the optical properties of this uniquely produced material.
“You can make lenses just like you would in a lens-making factory using regular glass,” she said. “What’s different about our bacteria is that they have very different and advantageous properties. To start, it’s very environmentally friendly to make microlenses this way compared to the way we make industrial glass. You don’t have to use super-hot temperatures or extreme low pressures. You don’t have to use a nano-laboratory or expensive clean room equipment.”
Discover the Benefits of ASME Membership
In addition, the bacteria-produced microlenses are much smaller than those currently produced in industry—and boast properties that could be used for specialized sensing applications in the future.
“Our bacteria make lenses that are 10 to 100 times smaller than what’s conventionally produced in factories,” she said. “That means, you could potentially make a lot of these tiny lenses and use them in different ways. You might array them on a surface for different sensing capabilities. And because our lenses are so small, that array could be really thin. You could have a cellular phone camera lens that’s even thinner than it is now.”
Producing these bacterial microlenses should also be easy to scale up in the future, Meyer added.
“There are a lot of people who have already worked out upscaling systems,” she said. “We usually make half a liter in our lab for testing purposes. But people have made fermenters where you can make 10 liters, a hundred liters or even more. I would imagine it would be fairly straightforward to create the same conditions as we do in our little flask in the lab.”
While there is still more work to be done, Meyer believes this is one of the first projects that reengineers organisms to have new optical properties. She hopes it will inspire others to continue innovating.
“This is a new way of having a living material at the single cell level. It’s just a little cell, with a little shell, made from one single enzyme,” she said. “People can now think creatively about sorts of applications that these kinds of optical properties could be useful for—it gives us an opportunity to think about how to use further engineering approaches to have different kinds of living lenses that are appropriate for different applications in the future.”
Kayt Sukel is a technology writer and author in Houston.
“I had never heard of [these sponges] before. But the idea that they could make this amazing, mineralized glass material and do it with one single enzyme called silicatein was very inspiring,” she said.
There are many interesting materials that exist in nature that Meyer would love to make using a genetic engineering approach. Yet, most of those materials are quite complex, requiring multiple enzymes and tissues to work together, sometimes in rather mysterious ways, to create the end product. After learning about the sea sponges and the single enzyme required to make silica, she thought it would be an interesting test case for a genetically engineered material—and the intricate glass produced, she hypothesized, could be leveraged to make microlenses, or lenses just a few micrometers in size, with the optical power to capture, focus, and manipulate light.
“It seemed to me that this would make an excellent synthetic biology project,” Meyer said. “And I wondered if it was something it would be possible to engineer bacteria to do.”
Current microlenses are only a few microns in size. They have the power to focus light on a microscopic scale, allowing precise manipulation of beams to support high-resolution imaging for camera sensors, fiber optics, and medical devices.
To see whether they could genetically engineer a biological microlens, Meyer recruited colleagues from the University of Colorado-Boulder, Delft University of Technology, and Leiden University, with collected expertise in optics, physics, and chemistry.
More for You: Big Promise for Largest Metalens
“We used a variety of E. coli that’s not a pathogen and doesn’t cause diseases or have toxic effects,” she said. “And we used it because this is a type of bacteria that is very well studied and characterized. There are a lot of genetic tools already available you can use to express new enzymes and new genetic parts in different parts of the cell.”
After conducting physics modeling in computer simulations, the group determined, in theory, engineering something the size and shape of the bacteria should produce a distinct silica shell with lens-type behaviors. With those tests to guide them, the team was inspired to bring this novel idea into the real world. They grew the engineered cells and checked for the presence of silica on each by analyzing each bacteria’s chemical properties. They then created mathematical models to better understand the optical properties of this uniquely produced material.
“You can make lenses just like you would in a lens-making factory using regular glass,” she said. “What’s different about our bacteria is that they have very different and advantageous properties. To start, it’s very environmentally friendly to make microlenses this way compared to the way we make industrial glass. You don’t have to use super-hot temperatures or extreme low pressures. You don’t have to use a nano-laboratory or expensive clean room equipment.”
Discover the Benefits of ASME Membership
In addition, the bacteria-produced microlenses are much smaller than those currently produced in industry—and boast properties that could be used for specialized sensing applications in the future.
“Our bacteria make lenses that are 10 to 100 times smaller than what’s conventionally produced in factories,” she said. “That means, you could potentially make a lot of these tiny lenses and use them in different ways. You might array them on a surface for different sensing capabilities. And because our lenses are so small, that array could be really thin. You could have a cellular phone camera lens that’s even thinner than it is now.”
Producing these bacterial microlenses should also be easy to scale up in the future, Meyer added.
“There are a lot of people who have already worked out upscaling systems,” she said. “We usually make half a liter in our lab for testing purposes. But people have made fermenters where you can make 10 liters, a hundred liters or even more. I would imagine it would be fairly straightforward to create the same conditions as we do in our little flask in the lab.”
While there is still more work to be done, Meyer believes this is one of the first projects that reengineers organisms to have new optical properties. She hopes it will inspire others to continue innovating.
“This is a new way of having a living material at the single cell level. It’s just a little cell, with a little shell, made from one single enzyme,” she said. “People can now think creatively about sorts of applications that these kinds of optical properties could be useful for—it gives us an opportunity to think about how to use further engineering approaches to have different kinds of living lenses that are appropriate for different applications in the future.”
Kayt Sukel is a technology writer and author in Houston.