Engineering Ways to Improve Electrical Grid Resilience
Engineering Ways to Improve Electrical Grid Resilience
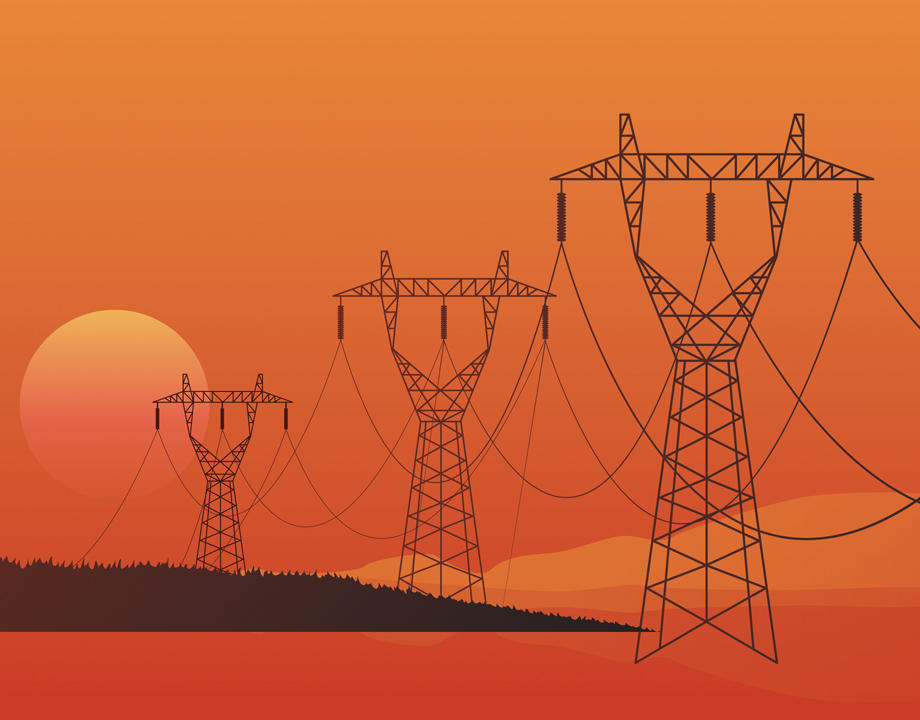
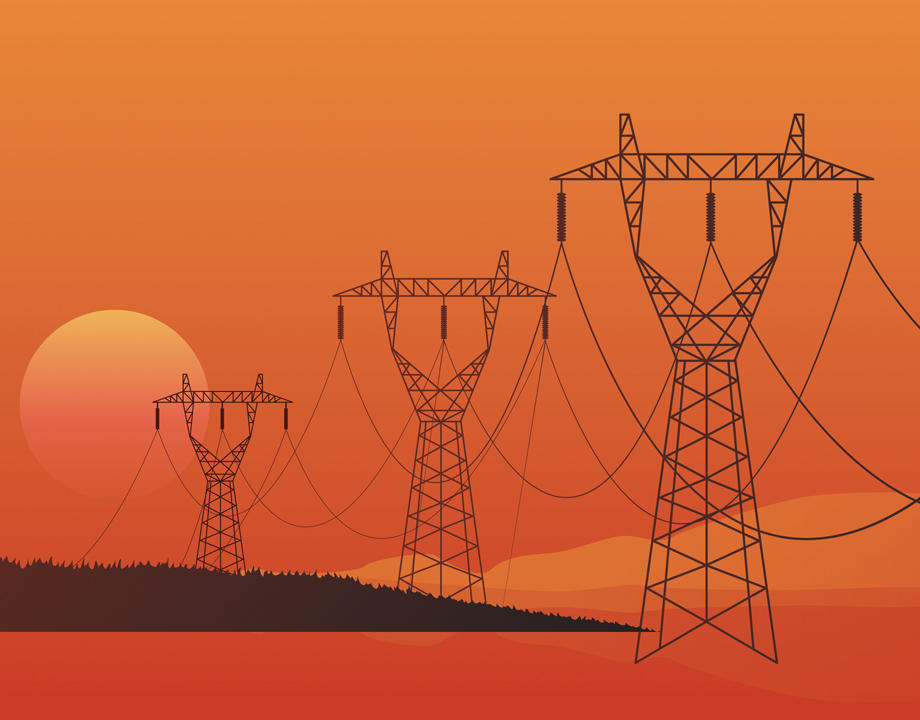
Utilities have lots of practice repairing lines downed by fallen trees. But when widespread or unusual calamities strike, they look to ways to make the grid less likely to break.
Under normal circumstances, an electrical outage is not a crisis. The power goes out—say, after a thunderstorm—and utility crews are on the job within hours to restore service and get the lights back on. Most electric utilities in this country have a reputation for reliability and recovering from situations like this.
But what if it’s a major hurricane? Hurricane Katrina devastated New Orleans and southern Louisiana in 2005, and Sandy in 2012 blacked out parts of New York City for days. More recently, the prospect of the coronavirus pandemic has focused electric grid operators on how to handle widespread personnel disruptions.
For decades, reliability has been the watchword for electric utilities, but now there’s a focus on a related concept: Resilience. It has gained notice as planners began thinking about increased natural disasters brought on by climate change, man-made interference due to malicious cyberattacks, and the instability brought about by adding large quantities of renewable energy. Resilience has become a legitimate field of study involving industry, academia, and government labs, complete with experts in the field.
According to Mike Bryson, senior vice president for operations at PJM Interconnection, a regional grid operator based in Valley Forge, Pa., “The concept of resilience involves protecting against multiple scenarios that are beyond reliability criteria.” These are very low frequency events that have high impact on society.
Whereas maintaining reliability involves dealing with discrete failures as they occur—think of the downed power lines after a storm—Bryson sees resilience as the means to take on multiple, simultaneous challenges to the grid. As an example, he recalls that in 2013, a team of gunmen used rifles to attack the Metcalf Transmission Substation near San Jose, Calif. The attackers damaged 17 transformers and cut off the data link to the substation to isolate it. According to reports, the damage took weeks to repair, at a cost of $15 million.
“What if they did that multiple places on the power grid at the same time?” Bryson asked.
The key, electric utility workers and university and government researchers agree, is to be proactive, rather than reactive. For instance, PJM began anticipating the moves it would need to make to protect control room dispatchers from a potential coronavirus outbreak even before the first cases were reported in the United States.
“We took a number of measures to protect the campus beginning in January,” the company said in a press statement, “and we had a telecommunicating plan in place that we successfully tested on March 13. At that time we determined to have most staff work from home, with critical operations staff and security on campus.”
The resiliency of the electric grid is a concept that still doesn’t have a hard-and-fast definition. The Electric Power Research Institute describes resiliency as involving prevention of damage, rapid recovery after an event, and survivability, or “the ability to maintain some basic level of electrical functionality to individual consumers or communities in the event of a complete loss of electrical service from the distribution system.”
Recommended for You: Using AI to Manage the Grid
The kinds of challenges that utilities and grid operators are contemplating have increased in severity over the past decade. Forget the simple ice storm, such as one in 2015 that knocked out power to 1.5 million customers in the U.S. and Canada. A report published by Lawrence Berkeley Laboratory in 2019 discussed a spectrum of challenges from simple reliability challenges such as trees falling on a local distribution circuit to so-called “Black Sky Events”—catastrophic, regional electric outages that might “include a coordinated cyber and physical terrorist attack, electromagnetic pulse event, geomagnetic disturbance, a widespread earthquake or a widespread extreme storm.”
Craig Rieger, P.E., chief control systems research engineer and a directorate fellow at the Idaho National Laboratory said, “When you start looking at resilience, you think of cyber attacks with a human involved. But there are multiple aspects to resilience. Some are benign; some are active. You’re looking to maintain that normalcy.”
As Bryson sees it, the resilience movement has evolved this century. “If you think of some of the natural disasters that we had in this country over the past 20 or 25 years, it really started to form then,” he said. “Think of Katrina and Sandy the multiple hurricanes that hit Puerto Rico. We’re pretty good at handling storms, but what if we get several storms in succession?”
But Rieger thinks it may go further back, saying resilience started as a research area back in the 1970s. “It focused historically on control systems. It involved things like set points and was human-controlled. SCADA (supervisory control and data acquisition) is an example. It can be used to collect data points from the grid and monitor power load changes,” Rieger said. “In the 1990s, it started including human aspects and has covered infrastructure the last 20 years.”
Rieger represents a relatively new breed of engineers specializing in resilience. At INL, he leads multidisciplinary research in the area of next-generation resilient control systems. His doctoral dissertation focused on measurements and control, with application to intelligent, supervisory ventilation controls for critical infrastructure.
Rieger and his INL colleagues began the International Symposium on Resilient Control Systems in 2008, working with nine universities on a program exploring issues related to threats to infrastructure.
“It started ‘researchy’ and was very academic,” Rieger said.
That initial program has expanded into the annual Resilience Week Symposium, adding industry representatives and a wider focus on critical infrastructure and communities. “We now focus less on technology,” he said. “It’s a friendly atmosphere where we have a dialogue.”
INL has created an Instrumentation, Controls, and Intelligent Systems signature, an area centered on developing systems for any application that requires monitoring, control, and human interaction. ICIS has a multidisciplinary team of some 50 scientists and engineers who specialize in resilient control systems, sensors, intelligent automation, robotics and intelligent systems, and human-system integration.
“I got into resilience as I was seeing many of the human, cyber, and control system challenges that would benefit from fundamental change,” Rieger said. The mantra at INL has begun to shift focus from reactive to proactive control of plants and incorporate mechanisms from the design phase through implementation of resilient control systems.
Outages, Large and Small
According to a 2017 study by the Rhodium Group, the average residential electricity customer experiences one to two outages a year. Most of these are small-scale; typically power is restored in little more than two hours, absent some major event. But major events play an outsized role in the statistics. From 2012 to 2016, the widespread blackouts from a single event—Hurricane Sandy—caused nearly 32 percent of the service disruption, measured in customer-hours.
Individual utilities are responsible for the distribution lines going to customers, while regional transmission organizations and independent system operators supervise competitive electricity markets and manage the high-voltage electricity grid to ensure reliability. They're also responsible for planning and designing the system up to 15 years in the future.
At PJM Interconnection, the regional transmission organization that coordinates the movement of wholesale electricity in 13 mid-Atlantic and Midwestern states and the District of Columbia, Bryson oversees resilience initiatives. PJM began taking some specific steps after the Federal Energy Regulatory Commission (FERC) released an administrative proceeding to study and better define grid resilience by engaging the system operators.
Bryson was involved in PJM’s fuel security initiative. A comprehensive study looked for vulnerabilities to generators’ fuel delivery systems, the corresponding effects on capacity availability, and the overall impact on system reliability.
“The fuel mix is changing dramatically, and you have a couple of different phenomena,” Bryson said. “One is that the amount of coal is reducing, and the amount of natural gas is increasing. That’s been a combination of the natural gas coming from the gas shale formation in Pennsylvania and Ohio, gas being very inexpensive.”
Watch a Video: Emerging Technology and the Electrical Grid
How else can you improve the resilience of an electrical grid? Bryson offers an example: “With substations, one way to increase resilience is to find ways around it so a failure becomes less critical. You could have a substation with six high-voltage lines coming in. If you can route two of those to another substation, you now can take it off the critical list. It doesn't have that same vulnerability; you spread it across the system.”
PJM has recently undertaken this as an initiative and plans to start construction on substations soon.
The academic world is contributing to resiliency measures, too. At North Carolina State University, the Future Renewable Electric Energy Delivery and Management (or FREEDM) Systems Engineering Research Center was created through funding from the National Science Foundation in 2008 to modernize the electrical grid to accommodate sustainable energy, such as wind and solar power. It has several industry members and collaborates with other universities including Arizona State, Florida A&M, Florida State, and Missouri University of Science and Technology.
The FREEDM Center has been involved in developing online tools for assessing vulnerabilities. The center has developed a platform to address cyber-physical security called Distributed Grid Intelligence, an effort led by Bruce McMillin of Missouri University of Science and Technology. And the Resilient Information Architecture Platform for Smart Grid, a collaboration with Gabor Karsair of Vanderbilt University that was funded via ARPA-E, is an open source software platform to develop, deploy, and run smart microgrid control algorithms.
The hope is that smart microgrids, with sensors embedded throughout the system, might be more resistant to failure and easier to bring back online than large, multi-state electric grids. But the emerging smart grid, together with distributed renewable energy such as rooftop solar, presents a new set of challenges to resilience.
“The smart grid involves more distributed energy down to the home level. That kind of penetration adds a level of vulnerability of a cyber threat. We certainly have to pay attention to that as the grid gets smarter,” Bryson said.
“You have the increasing penetration in the PJM region of wind and solar and other distributed energy resources,” he continued. “We’re looking at the reliability aspects. Part of it is the intermittent nature of renewables.”
As another factor, renewable energy typically flows in the reverse direction from conventional power, something for which the grid wasn’t designed.
According to Iqbal Husain, director of the FREEDM Center and a professor of electrical engineering at North Carolina State University in Raleigh, “The load always has to match the generation. So if we have a lot of renewable energy, one problem would be during midday with a lot of renewable energy and excess power generated by the PV, but there’s not enough load to take it, so that could cause instability and bring down the whole system. That has happened in Hawaii and other places.”
The FREEDM Center has been looking at hardware, too. The center has been developing a solid-state transformer (SST) as a replacement for the conventional transformers seen on pole tops and at substations.
“It has all the functionalities of a regular transformer stepping up or down, but in addition, it has electronics that allow us to control the power flow,” Husain said. “An SST can increase resilience of the electrical grid through its power and energy flow control features as well as its protection and coordination features through intelligence and peer-to-peer communications.”
Mainly, though, SSTs can level out renewable energy, Husain said. “Solid-state transformers can distribute the energy in a controlled way. But you also need to have storage capabilities like battery storage.” The SST can take power from excess energy generation and put it in storage and then release it when there is more load and less renewable energy, such as in the early evenings.
“The solid-state transformer can manage that power distribution so there’s always a balance between the load and generation,” Husain said.
Low Frequency, High Impact
Besides natural disasters, cyberattacks, and renewable energy, several other less-visible factors can affect grid resilience. “An example of an aspect most people don’t think about is the water used to fill boilers at power plants,” Rieger said. “You have to have a water supply. Some people model these dependencies.”
According to Bryson, another one being worked on is geomagnetic disturbance.
“The sun has the ability to cause disruption to the power system, and it happens fairly frequently. How do we update the system to make sure it can withstand solar flares?”
Electromagnetic pulses are another issue. “A rogue nation can detonate a nuclear device in the United States and take out portions of the grid.”
One recent resiliency test for electric grid operators has been the ongoing coronavirus pandemic.
Bryson was appointed PJM’s point person for handling the crisis. He sees this as a concrete example of a low-frequency, high-impact event. For more than a decade, he said, the North American Electric Reliability Council, which has authority from FERC, has encouraged regional transmission organizations and independent system operators to prepare for such events.
Editor's Pick: Preparing the Grid to Deal with the Heat
PJM has had a communicable disease and pandemic preparedness plan in place since 2006. Part of that plan is to have two separate control rooms, each functioning in parallel.
In New York State, which has been especially hard hit by the coronavirus pandemic, 37 workers of the state’s independent system operator volunteered to sequester themselves at two electric grid control centers. The 33 grid operators and managers, two facilities workers, and two food service staff members were living in trailers without contact with the outside world in order to maintain their health—and the stability of the state electric grid.
Like many resilience-oriented preparations, the steps seem like a lot of work for a far-fetched situation—until they are called upon in desperate hours to keep the lights on.
COMSOL Sidebar: Advanced Models Ensure A Resilient Grid
Managing the electrical grid, ensuring that it matches demand throughout the day, and keeping voltage and frequency within acceptable limits are fundamental to safety, reliability, and resilience. National Grid manages the high-voltage transmission network in England and Wales. Simulation software helps it to understand the impact of surrounding soil, cable age, repairs, and how the proximity of other cables affects the performance of a given section of buried transmission line.
Engineers at National Grid collaborated with the Tony Davies High Voltage Laboratory at the University of Southampton in modeling different cable components. Using finite element analysis with the COMSOL Multiphysics® software they have gained a better understanding of cable performance in response to environmental change and condition.
Focusing primarily on heat transfer, they first validated the ratings of various cable families, and then began to analyze capability at specific environmental pinch points. For example, when soil is wet, heat dissipates relatively quickly, while dry soil is more resistant due to the presence of small air pockets. The team accounts for soil dryness and cracking when modeling the trench in which a cable runs.
“There are standards for soil and specialized backfill materials that we populate in the model. Soil can vary enormously, so one has to adopt a relatively pessimistic assumption of how it will affect a cable to protect it for 60 plus years,” explained David Scott, an asset lifecycle engineer at National Grid’s Engineering & Asset Management department.
Another challenge is the availability of spares for maintaining older systems and repairs that result in mixed materials. “Many older cables include a lead outer sheath, whereas new cables tend to be aluminum. If we need to do repairs we prefer to replace only the damaged section because of the obvious cost implications,” said Scott. “Existing industrial standards do not consider the case of mixed conductors in parallel. COMSOL allows us to calculate cable circuit losses and understand what countermeasures are required when specific materials are combined.”
Advanced simulation software enables Scott and his colleagues to work more efficiently. “It is easy to focus on the physical problem without getting caught up in mathematical complexities,” he said. “If we ensure accurate input, the simulation has proven extremely reliable and helps us to make good decisions about cable laying and repairs.”
Tom Gibson, P.E. is a consulting mechanical engineer based in Milton, Pa., who specializes in machine design, green building, and solid waste. He publishes Progressive Engineer, an online magazine.
Explore more on grid resiliency in our Energy Special Report 2020